Proteins

C4 Streptavidin Complex

C5 Symmetry

C6 Symmetry

D2 Symmetry

D3 Symmetry
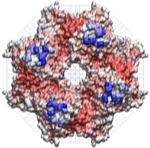
D4 Symmetry
Why Protein-Based Nanotechnology?
A key requirement for the practical realization of nanotechnology is a suitably flexible and scalable manufacturing process for nano-scale devices. In the context of engineered nanostructures, there are two different aspects of scalability. The first aspect involves the ability to manufacture structures using a parallel manufacturing process.
The unique self-assembly properties of protein molecules directly address this fundamental issue. Proteins, which are polymers of amino acids, spontaneously fold to form uniquely organized, tightly packed structures that self-organize thousands of atoms with atomic precision over ranges of 1 to 100 nm, and manifest a wide range of structural, catalytic, binding, and signal transducing properties. Many living organisms exist in extreme environments, so that their proteins have naturally evolved to maintain structural stability at elevated temperatures. Proteins can be engineered to bind to surfaces with a defined orientation, a characteristic that is important in processes such as active filtration, directional transport of chemical substances, or charge storage on surfaces. The rich functionally of proteins physically arises from near equivalence of several different types of interaction forces (e.g. electrostatic, van der Waals interaction, and solvation effects) and the corresponding effects of statistical energy fluctuations on the order of a few kT that dominate the behavior of biological systems on the molecular scale (Philips &Quake)
By engineering surfaces on which only a few reactive atoms are precisely positioned, and then using these sites for the specific attachment of self-assembled proteins, the possibility exists to create protein-based nanostructures of arbitrary complexity. This combination of top-down and bottom-up self assembly consequently offers the possibility of solving the basic problem of fabrication scalability for nanostructures.
A second aspect of scalability applies to the use of nanostructures for intrinsically large-scale applications. An example would an application like water purification or desalinization, which might utilize active membrane structures organized on the nanostructural level, but would have to be manufactured at very large scale to provide useful capacity. In this respect it is estimated that engineered proteins may ultimately be produced in green plants for a few dollars a pound, potentially making large scale applications economically feasible propositions.
A key requirement for the practical realization of nanotechnology is a suitably flexible and scalable manufacturing process for nano-scale devices. In the context of engineered nanostructures, there are two different aspects of scalability. The first aspect involves the ability to manufacture structures using a parallel manufacturing process.
The unique self-assembly properties of protein molecules directly address this fundamental issue. Proteins, which are polymers of amino acids, spontaneously fold to form uniquely organized, tightly packed structures that self-organize thousands of atoms with atomic precision over ranges of 1 to 100 nm, and manifest a wide range of structural, catalytic, binding, and signal transducing properties. Many living organisms exist in extreme environments, so that their proteins have naturally evolved to maintain structural stability at elevated temperatures. Proteins can be engineered to bind to surfaces with a defined orientation, a characteristic that is important in processes such as active filtration, directional transport of chemical substances, or charge storage on surfaces. The rich functionally of proteins physically arises from near equivalence of several different types of interaction forces (e.g. electrostatic, van der Waals interaction, and solvation effects) and the corresponding effects of statistical energy fluctuations on the order of a few kT that dominate the behavior of biological systems on the molecular scale (Philips &Quake)
By engineering surfaces on which only a few reactive atoms are precisely positioned, and then using these sites for the specific attachment of self-assembled proteins, the possibility exists to create protein-based nanostructures of arbitrary complexity. This combination of top-down and bottom-up self assembly consequently offers the possibility of solving the basic problem of fabrication scalability for nanostructures.
A second aspect of scalability applies to the use of nanostructures for intrinsically large-scale applications. An example would an application like water purification or desalinization, which might utilize active membrane structures organized on the nanostructural level, but would have to be manufactured at very large scale to provide useful capacity. In this respect it is estimated that engineered proteins may ultimately be produced in green plants for a few dollars a pound, potentially making large scale applications economically feasible propositions.